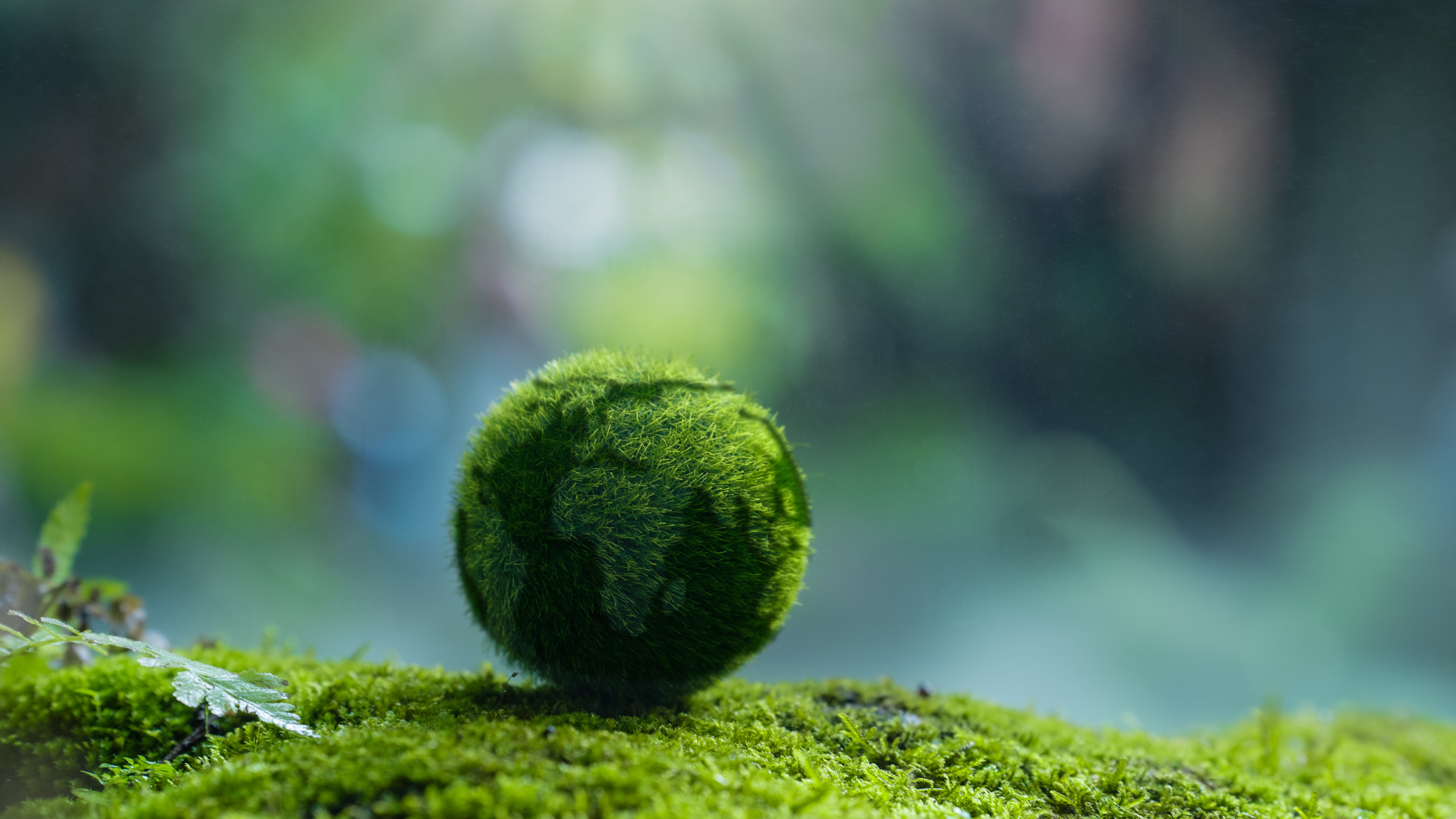
Organometallics
CO2 activation and binding
CO2 activation and binding
Organometallic chemistry has emerged as a pivotal field in modern research due to its ability to bridge the gap between organic and inorganic chemistry, facilitating novel catalytic processes and sustainable applications. One of the most compelling areas of study within this domain is the activation and binding of carbon dioxide (CO₂). As a readily available, abundant, and relatively inert molecule, CO₂ presents both a challenge and an opportunity in the quest for green chemistry solutions. The activation of CO₂ using organometallic complexes can unlock pathways to its conversion into valuable chemicals and fuels, offering promising routes for carbon capture and utilization (CCU). Understanding the mechanisms and interactions involved in CO₂ binding to metal centers is crucial for developing efficient catalysts that drive this transformation forward.
1. CO2 activation and binding:
a) Strong CO2 interaction: Organometallic complexes usually have metal centres which can strongly interact with CO2 which enables both the activation and binding of CO2 molecules. This is one of the essential features required for capturing CO2 and then converting it into more stable or valuable forms.
b) Variety of binding modes: There are a number of ways in which CO2 can coordinate well with the metal centre including bent or linear binding depending on the complex. This versatility in binding modes enhances the efficiency of CO2 capture.
2. Chemical tunability:
a) Ligand modification: It is possible to tune the properties of the organometallic complexes by modifying the ligands attached to the metal centre. This allows for the design of complexes with specific affinities for CO2 or tailored reactivity for CO2 conversion.
b) Tailored electronic properties: The process of CO2 capture and activation can be optimized by adjusting the electronic properties of the complex which is done by choosing different suitable metals and ligands. This tunability plays an essential role in developing highly efficient CCS systems.
3. Catalytic activity:
a) Catalysis for CO2 conversion: Most of the organometallic complexes act as catalysts, smoothing the process of chemical transformation of captured CO2 into different useful compounds like methanol, formic acid or carbonates. This capability to integrate capture with chemical conversion is one of the most significant advantages in CCS applications.
b) Energy-efficient processes: The catalytic activity of organometallic complexes can minimize the energy barriers for CO2 conversion reactions, making the processes more energy-efficient and potentially more economically viable.
4. Selectivity for CO2:
a) High selectivity: High selectivity is one of the factors essential for capturing CO2 gas from mixed gas streams. Hence, several organometallic complexes can be designed which can selectively capture CO2 over other gases like N2 or O2, commonly found in industrial emissions.
b) Specific interaction mechanisms: The specific electronic interactions between the metal centre and CO2 can be fine-tuned to enhance selectivity, ensuring that the complex preferentially binds CO2 even in the presence of other gases.
5. Structural versatility:
a) Diverse structures: It is possible to synthesize a wide range of structures, from simple mononuclear complexes to highly complex multinuclear or metal cluster systems. This structural diversity allows for the investigation of different mechanisms and efficiencies for CO2 capture and conversion.
b) Multifunctional complexes: Some organometallic complexes can simultaneously capture CO2 as well as facilitate its conversion or storage, being multifunctional in nature, thereby simplifying the overall carbon capture and storage (CCS) process.
6. Reversibility of CO2 binding:
a) Reversible capture: Some organometallic complexes can reversibly bind and release CO2, making them ideal for cyclic processes wherein CO2 is captured and then released for storage or utilization. This reversibility plays an important role for various practical applications, where the material needs to be regenerated without significant loss of efficiency.
b) Mild conditions for release: Most importantly, the process of release of CO2 from organometallic complexes occurs under relatively mild conditions like low temperature or pressure changes, which further aids in reducing the energy required for the regeneration process.
7. Durability and stability:
a) Thermal and chemical stability: The organometallic complexes are designed in a way that they remain stable under different conditions required for both CO2 capture and conversion like high temperatures and the presence of other reactive species.
b) Resistance to degradation: Organometallic complexes find their utility in CCS applications as they remain stable and retain their activity over multiple cycles of capture and release.
8. Scalability and proccessability:
a) Synthetic accessibility: It is possible to synthesize the organometallic complexes using well-established methods, making them accessible for scaling up in industrial processes.
b) Integration with other materials: Lastly, the organometallic complexes can be integrated with other materials including porous supports or polymers in order to accelerate their performance in CCS applications. Hence, this hybrid approach can combine the strengths of different materials for more effective CO2 capture. Various organometallic complexes exhibit the adaptability across various energy sectors making them a valuable tool in supporting the transition to a more sustainable energy system.
Some aspects of the disclosure focus on how organometallic complexes can be employed for various energy applications using carbon capture and storage technique (CCS):
Synergistic CO2 utilization: In most of the nuclear power plants, the entrapped CO2 can be utilized for generating the synthetic fuels and chemicals with different organometallic complexes acting as catalysts for these reactions, thereby utilizing the heat and power generated by the reactor.
CO2-binding organometallic complexes: In several geothermal systems, where CO2 is used as a working fluid, organometallic complexes can be effectively utilized to capture CO2 before it is reinjected underground. This capture can occur in surface facilities where CO2 is separated from the geothermal fluid.
CO2 capture in ancillary processes: In nuclear plants, organometallic complexes can be used to capture CO2 from various ancillary processes like the production of materials or chemicals used in nuclear fuel processing or waste management.
Synthetic fuel production: Organometallic complexes can be employed in capturing CO2 and then further catalysing its conversion into various useful fuels such as dimethyl ether, methanol or other hydrocarbons.
Integration with electrolysis: Several organometallic complexes can be utilized along with the electrolysis that produce hydrogen from water. The captured CO2 is then combined with hydrogen to generate the synthetic fuels. Hence, CCS technique can be used for the production of sustainable fuels that can replace fossil fuels, contributing to the decarbonization of transportation and other sectors.
Closed-loop capture: Organometallic complexes enable a closed-loop system wherein CO2 is being captured, cycled and stored, thereby reducing emissions while maximizing the geothermal energy extraction.
Complex-assisted CO2 removal: Organometallic complexes can bind to CO2 selectively, hence can be used in capturing CO2 produced during the steam methane reforming and aerothermal reforming processes, thereby ensuring the production of high-purity hydrogen.
Uses:
1. Cement manufacturing: Organometallic complexes can be employed to capture CO2 emitted during the calcination process in cement production wherein these complexes can chemically bind to CO2 at high temperatures.
2. CO2 capture from blast furnaces: Most of the complexes can be used in capturing CO2 from the off-gases of blast furnaces, wherein large amounts of CO2 are produced during the process of reduction of iron ore.
3. Syngas purification: Organometallic complexes can be used to selectively capture CO2, allowing for the production of cleaner syngas which can be used in various chemical processes or energy production. As a result, the complexes employed in steel and iron manufacturing can minimize the carbon footprint of the industries.
4. 5. 6. 7. Fertilizer manufacturing: Urea can be directly synthesized by various organometallic complexes that capture CO2 during the Haber-Bosch process. These complexes enable the reaction between ammonia and CO2 to produce urea. Hence, the complexes can be designed to operate effectively under specific temperature and pressure conditions ensuring that CO2 is captured and utilized with minimal energy input. CO2 activation, conversion and process integration: Organometallic complexes can be used in order to capture CO2 followed by catalyzing its conversion into valuable chemicals like methanol, urea and formic acid. In several chemical manufacturing plants, these complexes can be integrated into existing processes to trap CO2 emissions and finally convert them into feedstocks for further production. This CCS technique hence enables to capture CO2 by converting it into commercially useful products thereby lowering the raw material costs. Plastic manufacturing: In several plastic manufacturing plants, reactors equipped with organometallic complexes can trap CO2 emissions and directly convert them into polymer precursors, integrating carbon capture and material production. Also, these complexes can capture CO2 and catalyse its polymerization into several useful biodegradable plastics or other polymeric materials. One of the major utility of CCS technique is to produce polycarbonates from the released CO2 emissions, thereby re.ducing the carbon footprint of plastic manufacturing. Glass manufacturing: Organometallic complexes can be used for capturing CO2 from exhaust gases of glass melting furnaces, where raw materials are heated to form glass. The complexes are specifically designed so that they can operate at high temperatures typical of glass production. Again, this CCS technique can be used for utilizing CO2 emissions in glass manufacturing process as it helps in reducing the carbon footprint of the industry, lowering the production costs through the creation of useful byproducts.
8. Textile and apparel manufacturing: In textile dyeing processes, wherein CO2 is being used as a solvent, the organometallic complexes can be integrated into various dyeing systems that use supercritical CO2 as a dye carrier followed by capturing and recycling CO2 for reuse. Hence, the process becomes more environment friendly and sustainable by reducing the water use and chemical wastes while lowering the carbon emissions after the CO2 capture.
9. Food and beverage manufacturing: Lastly, the organometallic complexes can be employed for capturing CO2 from various fermentation processes or food processing activities wherein the captured CO2 can be purified and reused in the carbonation of food preservation processes. In beverage manufacturing, a closed loop system can be generated where the CO2 produced during fermentation is captured, purified, reused and finally minimizes the need for externally sourced CO2.
Organometallic complexes that can be exploited for carbon capture and storage utility:
1. Metal-Carbon Dioxide Complexes:
a) Transition metal complexes: Various transition metals such as nickel, rhodium, ruthenium possesses the ability to form stable metal-CO2 adducts. These complexes in turn activate CO2 making it easier to convert into other chemicals.
b) Alkali and alkaline earth metal complexes: Metals such as magnesium and lithium also have the tendency to form complexes with CO2.
2. N-Heterocyclic Carbene (NHC) complexes: These complexes exhibit strong σ-donating properties which help in stabilizing metal-CO2 interactions, thereby enhancing the ability to capture and activate CO2.
3. Metalloporphyrin’s:
a) Iron and cobalt porphyrins: These complexes mimic natural enzymes (like carbonic anhydrase) which facilitates the process of conversion of CO2 into bicarbonate in biological systems.
4. Organometallic complexes with redox-active ligands:
a) Vanadium, manganese and chromium complexes: The ligands associated with these complexes have the ability to undergo reversible redox reactions, thereby providing a mechanism to capture and release CO2.
5. Carbene complexes: Fischer carbene complexes being characterized by the presence of a metal-carbon double bond have the ability to get coordinated with CO2 via the metal present. This interaction results in CO2 activation and also facilitates further chemical transformations.
6. 7. 8. Transition metal pincer complexes: Pincer ligands, with their robust chelating ability aids in stabilizing the metal centres present in unusual oxidation states, thereby making them effective in capturing and converting CO2. Transition metal carbonyl complexes: Metal carbonyl complexes undergo reactions with CO2, resulting in the formation of new carbon-containing products which are being further studied for their potential in catalytic CO2 reduction. Multi-metallic Clusters: Organometallic clusters with multiple metal centres provide cooperative interactions for enhanced CO2 binding and activation.